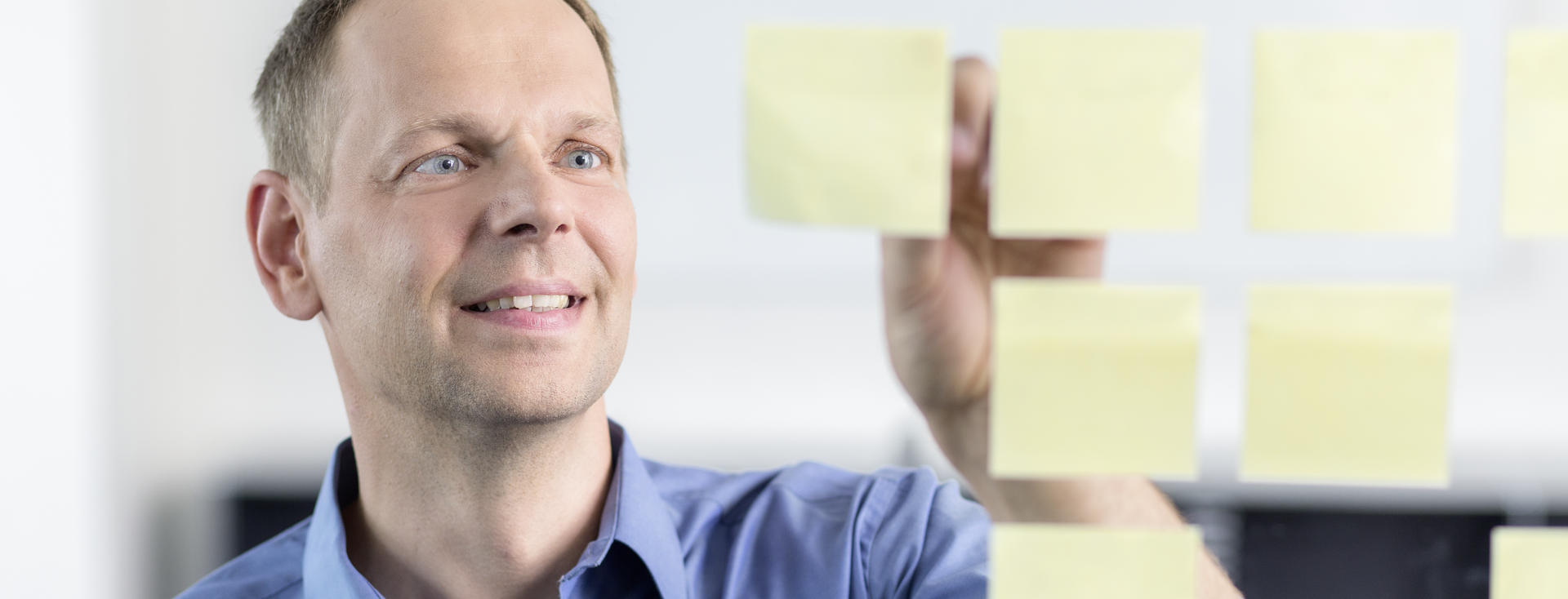
Development of a measuring method for characterizing the surface of Pressure Sensitive Adhesives (PSA)
Hydrophobic Recovery and Wilhelmy measuring method with acrylic-acid-based products
The characterization of the surface properties of Pressure Sensitive Adhesives (PSA) is an important part of the product development and optimization. Variable concentrations of the acrylic acid content enable the surface polarity to be adjusted to suit the particular substrate. We have evaluated tensiometric contact angle measurement as an alternative to the optical sessile drop method based on acrylate-based PSAs with different acrylic acid content. This method was shown to be well suited and has advantages compared with the optical method. The dynamics of possible reorientation of the surface functional groups in the PSA were investigated as part of a long-term study of the water and diiodomethane contact angles of samples conditioned in water. Our results indicate that the timescale for such Hydrophobic Recovery lies in the range with an order of magnitude of less than one minute. The advancing angles with water show a linear relationship with the acrylic acid content of the PSA. Wilhelmy CA measurements are therefore well suited for adjusting changes to the surface polarity caused by the variable acrylic acid content.
Background
Tesa SE is one of the world’s leading producers of adhesive solutions for trade and industry, consumers and handicraft with 125 years’ experience in coating technology and the development of adhesive compounds and innovative products and solutions.
An accurate knowledge and characterization of the substrates to be glued are essential for the development of new Pressure Sensitive Adhesives (PSA). This necessity results from new trends and developments in low-surface-energy coatings (e.g. so-called Lotus effect, easy-to-clean and powder coatings) and surface treatments (e.g. corrosion protection of sheet metal with oil). In addition to the influence of viscoelastic properties of the adhesive compound, the adhesive force or peel resistance is significantly affected by the interaction and adhesion of the adhesive compound with the surface. In order that this may be taken into account in the development of PSA, knowledge of the surface tension and surface free energy of the adhesive compound and the substrate, respectively, is of advantage.
This report is concerned with the development of a method for determining the surface polarity of viscoelastic, flowable polymers. Polyacrylates in particular are investigated as these are frequently used in PSA for various applications and can be quickly adapted to different substrates by reorienting surface functional groups.
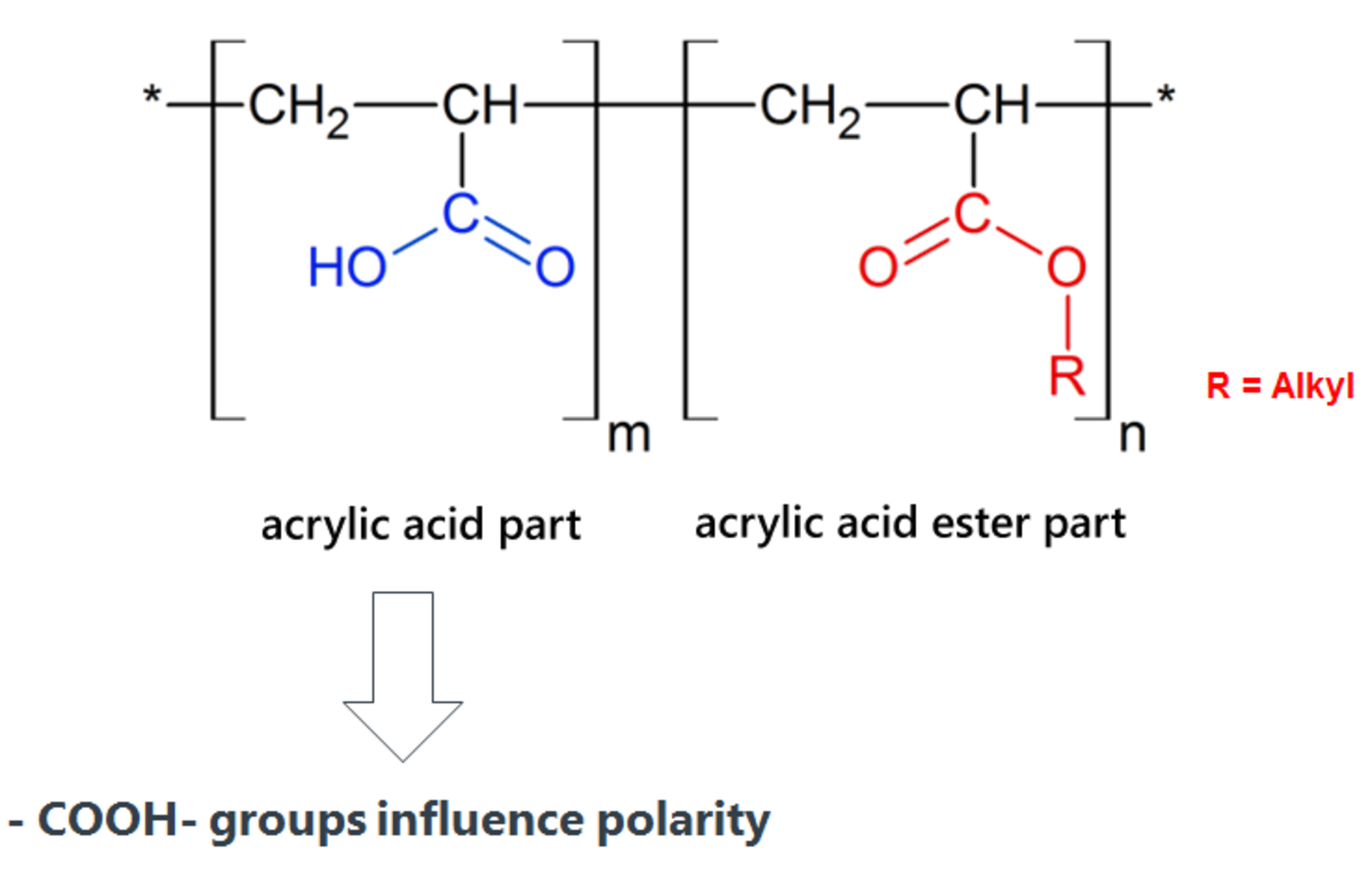
In the development of acrylic-acid-based PSA, along with the cohesion, it is desirable to adjust the surface polarity in an optimum manner for the particular substrate and applications by appropriate variation of the acrylic acid content.
Increasing the acrylic acid concentration increases the polarity of the polymer and, in particular, the proportion of functional groups which can be included in the bonding of hydrogen bridges with one another and also with the substrate. This process is to be checked by direct measurement of the surface polarity by means of the contact angle (CA).
The optical method of CA measurement using a sessile drop which is frequently used at present produces water CAs significantly greater than 90°. However, large water CAs of this kind are not consistent with the expected polar properties of acrylic acid in the PSA (see Fig. 1). Rather, the cause presumably lies in the fact that the adhesive compound molecules are flexible. If an adhesive layer is stored in air, the different side chains orientate themselves in such a way that the polar parts, for example, turn inwards (Hydrophobic Recovery). Due to the intra- and intermolecular interactions of the polar groups, these are often somewhat less mobile than the non-polar groups. From other polymers, it is also known that the CA hysteresis is generally affected by the orientation and mobility of different molecules at the interface [1, 2, 3].
On the one hand, this study was concerned with the question of how quickly this reorientation takes place.
On the other hand, it was to be investigated whether the CA correlates with the acrylic acid content as would actually be expected. In the past, such a correlation could not be clearly established with the help of optical CA measurements using the sessile drop method. For this reason, tensiometric CA measurement using the Wilhelmy method as an alternative was evaluated in this study. This method is often more precise than optical measurement and produces more comprehensive data with less effort and time. Whereas, strictly speaking, with the sessile drop measurement, the CA is determined at only two points, a single Wilhelmy measurement provides the CA averaged over a larger area based on a large number of measuring points. In this study, every single Wilhelmy CA measurement gave the advancing CA averaged over an area of 320 mm2 based on 80 individual measurements. The measurement assumes a regular (e.g. plate-like or cylindrical) sample shape, which limits the scope of this method, but was easily possible as part of this investigation.
As result of the gain in precision, it was to be established whether a possibly weaker correlation, which was merely hidden due to the scatter of optical CA measurements, existed between CA and acrylic acid content. However, at this juncture, we would like to point out that the basic findings, namely unusually large sessile drop CAs, were confirmed by comparably large Wilhelmy advancing angles.
Samples, preparation and measuring method
Polyacrylates with different acrylic acid concentration and otherwise identical comonomer composition were provided by tesa SE. The acrylic acid concentrations used corresponded to those which are normally used in adhesive compounds in order to cover as large a range of applications as possible.
The adhesive compounds were applied to both sides of cover slips and masked with siliconized release paper on both sides until the start of the experiments at KRÜSS.
Before measuring the CA, all samples were conditioned in water by suspending the cover slips in double distilled water for at least 72 h. Contact angles were then measured with water and diiodomethane (DIM) with different time intervals using the Wilhelmy plate method on a KRÜSS Force Tensiometer – K100. Two cover slips (samples) were investigated for each liquid and concentration. In addition, at least two cover slips for each concentration were measured immediately after removing the protective film.
The measuring speed for the dynamic CA measurements was 3 mm/min and the immersion depth was 8 mm for all measurements. Figure 2 shows a cover slip coated with a PSA having 12% acrylic acid content on a sample holder after a water CA measurement.
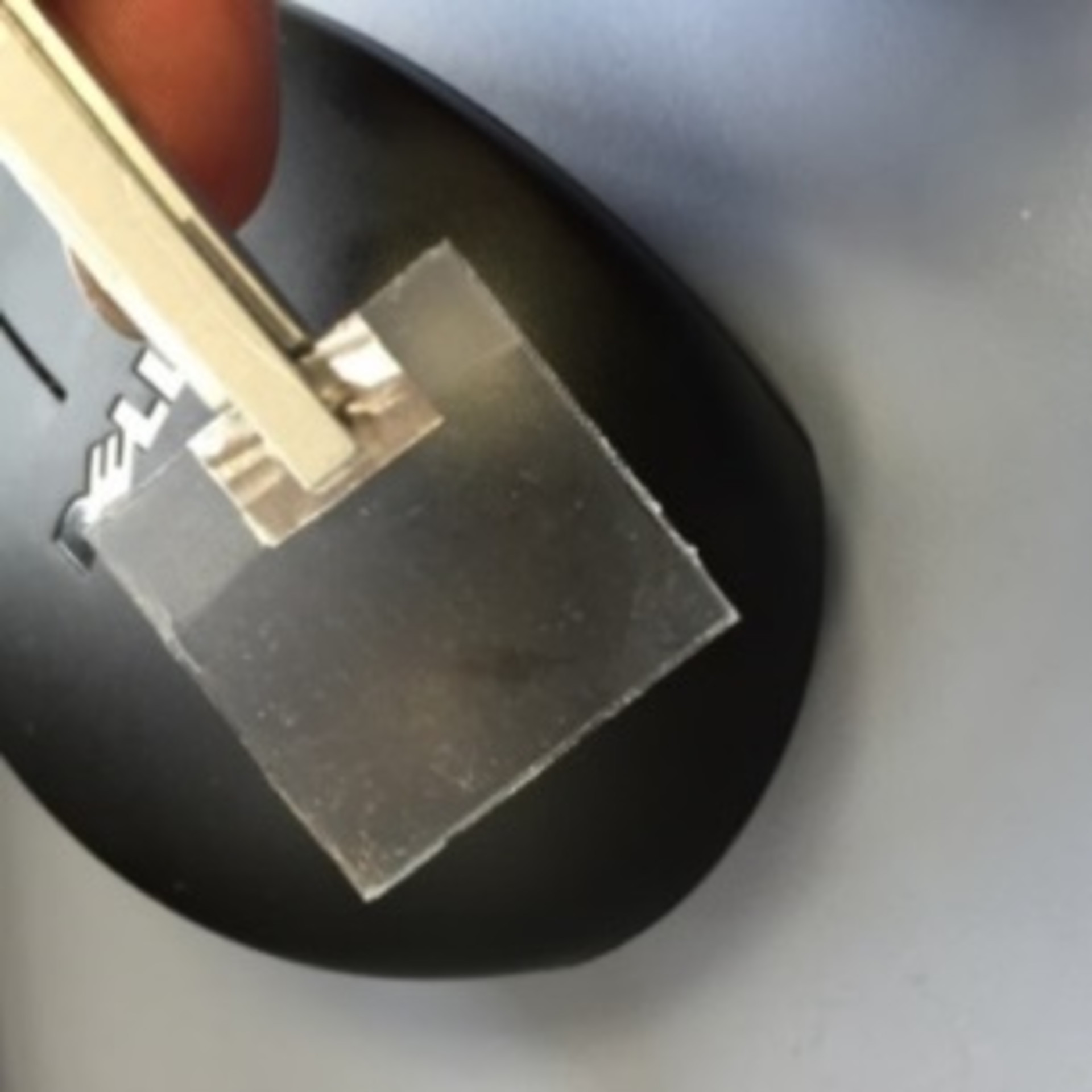
Optical water CA measurements were carried out on individual samples on the KRÜSS DSA100 using the sessile drop and captive bubble methods. In doing so, the drops and bubbles were placed using a needle dosing unit.
Results and discussion
Contact angle hysteresis
Figures 3a and 3b show by way of example the water and DIM contact angles as a function of the immersion depth for the PSAs with 1% and 15% acrylic acid content directly (less than one minute) after conditioning.
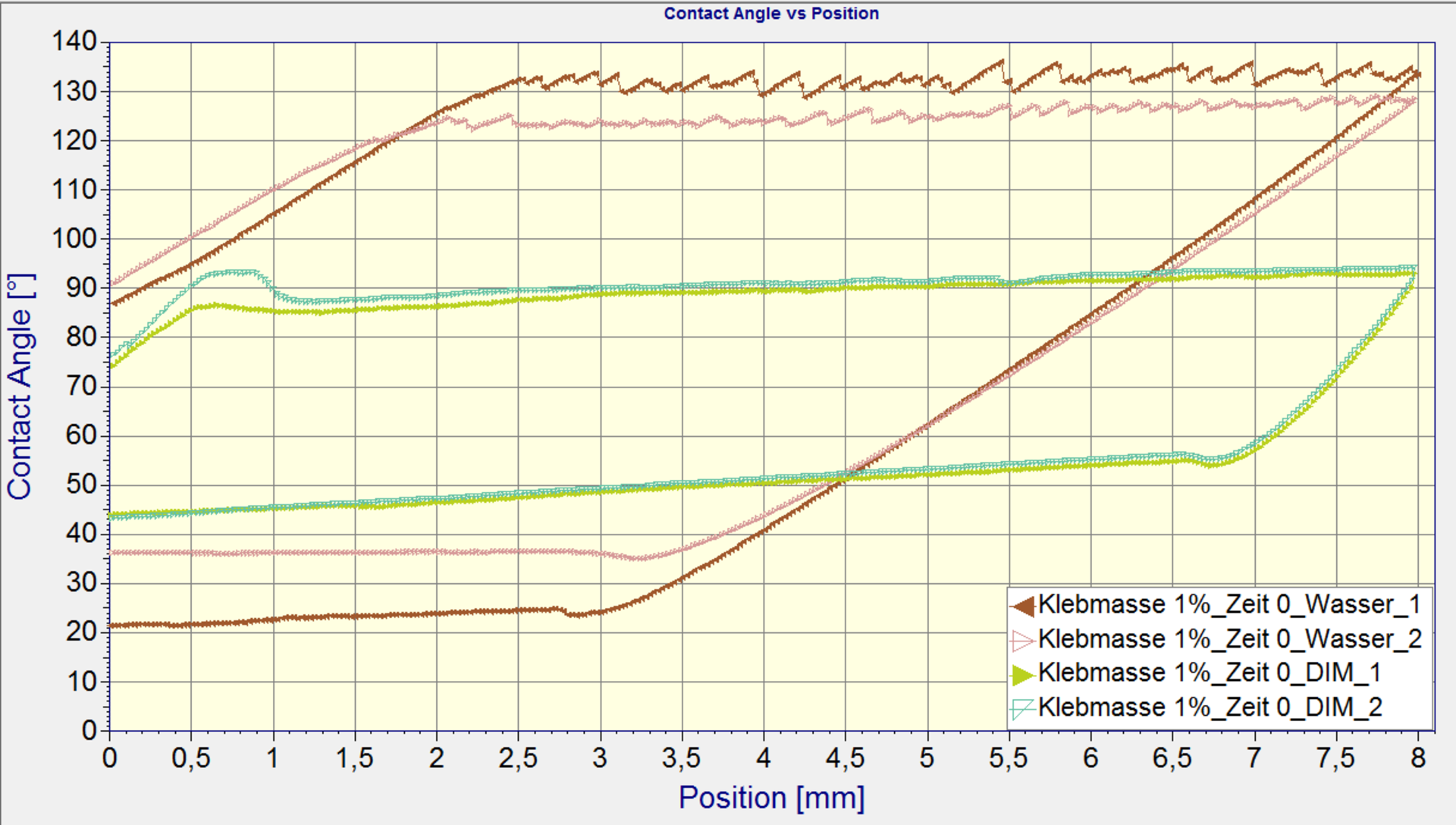
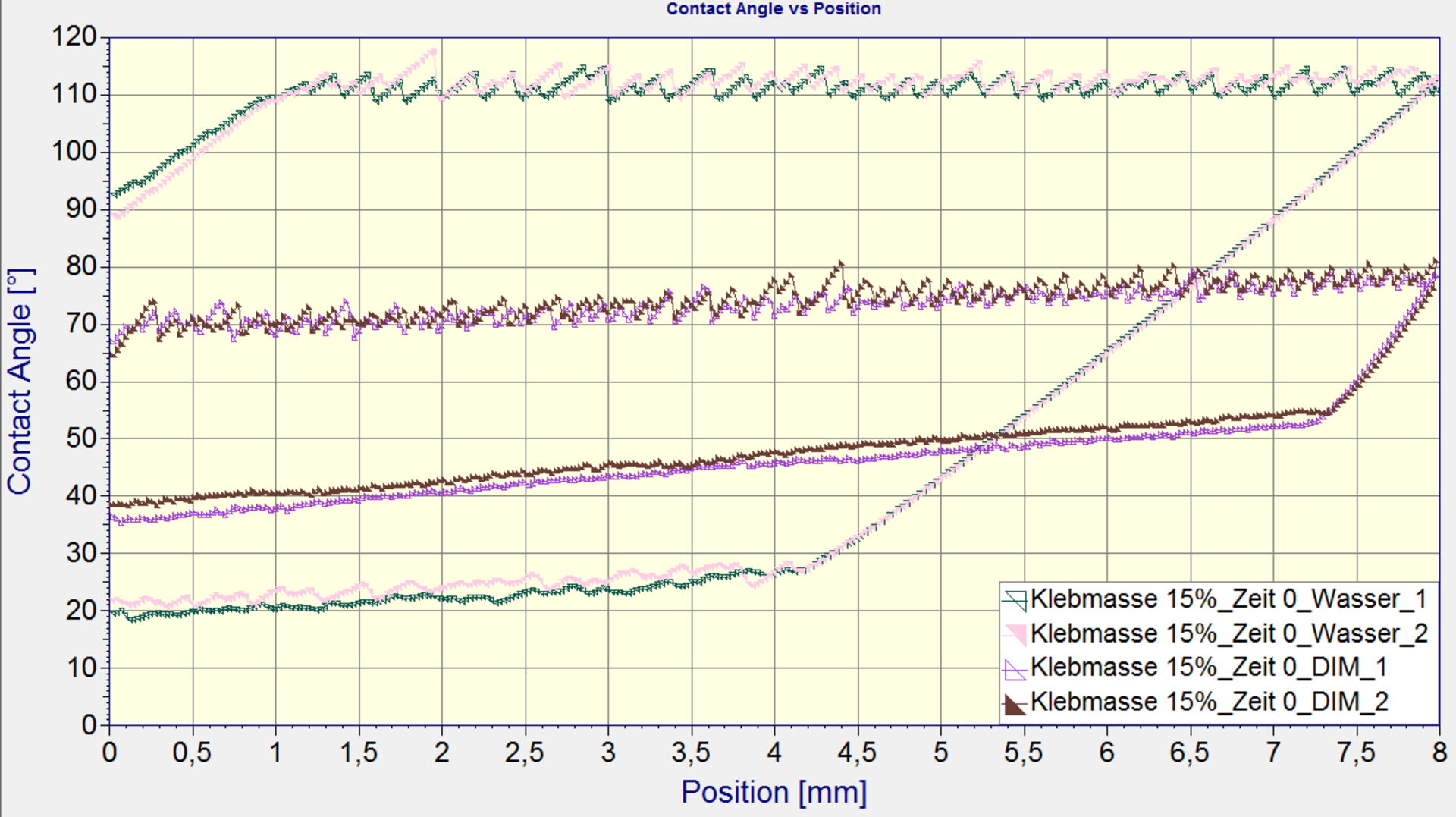
The reproducibility of the samples was good throughout, and particularly good for samples with larger acrylic acid content. Samples with smaller acrylic acid content were possibly less homogenous, as statistically polymers or oligomers without acrylic acid occur at an increasing rate. The greater variations in the measured CA can therefore be explained by an actual variation of the surface properties of different samples. However, further measurements would be necessary to reach a more reliable conclusion.
The sample-dependent variations of the water receding angle of approx. 15° for the 1% sample to be seen in Fig. 3a were the largest scatters observed. As a rule, the deviations were less than 3° (cf. Fig. 4).
The water advancing angle was 130° for the 1% PSA and 110° for the 15% PSA. The receding angles for both samples were 20°. The contact angle hysteresis for all measured acrylic acid concentrations was very large at more than 80°. This hysteresis can presumably be linked to a reorientation of the side chains. Before starting the advancing angle measurement, the sample with the PSA was suspended in the (fully dispersed) air and the polar side chains were turned into the samples. As a result, an initially non-polar surface was measured during insertion and the advancing angle was significantly greater than 90°. When the sample was withdrawn from the water during the receding angle measurement, the polar side chains had turned out of the sample due to polar interactions with water. As a result, the polarity of the surface free energy had greatly increased. When measuring the receding angle, the de-wetting behavior of water is therefore determined by a strongly polar surface. Accordingly, the measured receding angles are quite small and lie in the region of about 20°.
Although at approx. 30° the CA hysteresis for DIM is considerably smaller than for water and is comparable with the CA hysteresis on clean cover slips (~ 30-40°), it can still be easily measured. As no turning out or turning in of the side chains is to be expected in measurements with the totally non-polar DIM, this indicates that not only Hydrophobic Recovery, but also other effects (e.g. topological inhomogeneity) contribute to the CA hysteresis.
Stick-slip behavior and possible causes
All water advancing angle measurements show a sawtooth-like characteristic, a so-called stick-slip characteristic. This is less evident only with the 1% sample. For all other concentrations, it is comparatively clear and easily reproducible with regard to amplitude and period. Interestingly, the CA measurements with the fully non-polar test liquid DIM show a similar stick-slip characteristic. However, in the case of both water and DIM, this is shown only for the advancing angle and not for the receding angle measurements.
Without further investigations, a full explanation of this stick-slip characteristic remains speculative. The following four causes, for example, would be conceivable:
1. Topographically caused unevenness, which although it affects the advancing angle is smoothed out during the receding angle measurement. The question remains as to how this smoothing occurs.
2. A silicone surface transferred from the release paper to the PSA. As advancing angles are particularly sensitive to low-energy inhomogeneities and receding angles to high-energy inhomogeneities [4], this could explain why the stick-slip characteristic was only observed when measuring the advancing angle. Alternatively, it is also conceivable that such a silicone surface is washed off when measuring the advancing angle. However, such a silicone surface would presumably not lead to such a regular stick-slip characteristic as observed by us.
3. Static inhomogeneities, that is to say permanently present areas which are low in acrylic acid and rich in acrylic acid.
4. Dynamic inhomogeneities commensurate with a mechanism whereby, following the buildup of stress in the stick region, the abruptly proceeding front then passes over the still non-polar surface, which cannot reorientate itself in polarity quickly enough.
However, these or other possible causes were not investigated further in the course of the study.
Time-dependency of CA Hydrophobic Recovery
Figure 4 shows by way of example the water advancing angle measured on the 12% PSA samples as a function of time at the end of conditioning.
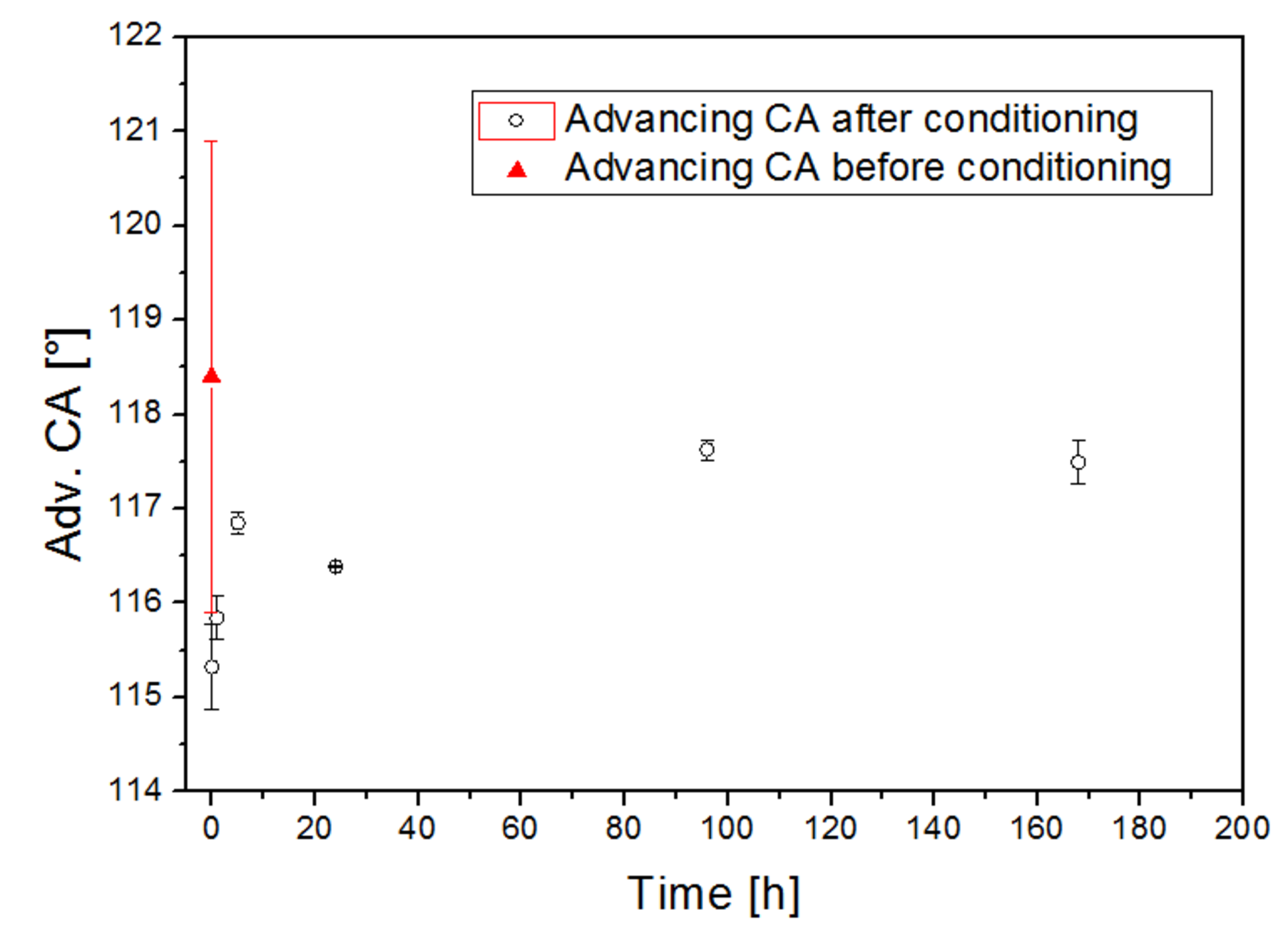
The initially somewhat greater scatter which occurs with unconditioned samples (shown in red) could evidently be reduced by pre-conditioning. A clear time-dependency was not observed; the changes which can be seen barely lie outside the scatter range. The water CA increases from 115.3° to just 117.6° over 160 h.
A single water CA measurement on a 12% PSA which was conditioned for 48 h shows an advancing angle of 116° and therefore no significant difference from the samples which were conditioned for a shorter time. The water CA measurements on the PSA with other concentrations also showed no obvious time-dependencies.
The DIM CA also appeared to be unaffected by preconditioning, i.e. the CAs measured before and after completion were the same within the limits of the measurement scatter and accordingly showed no time-dependency.
This and the fact that all water CAs were already significantly above 100° at time zero after preconditioning leads to the conclusion that the dynamics of a possible reorientation of side chains is substantially complete within a period of less than one minute. The timescale for any Hydrophobic Recovery which may occur is therefore of the order of magnitude of less than one minute. Possible drying effects within this timescale also have an equally insignificant effect on the measured CA. This is also supported in that the CAs measured directly after conditioning have values which are clearly too high for moist surfaces.
In order to investigate possible Hydrophobic Recovery further, optical CA measurements were also carried out on the 12% sample.
Figures 5 and 6 show by way of example water CA measurements on the 12% sample using the sessile drop and captive bubble methods.
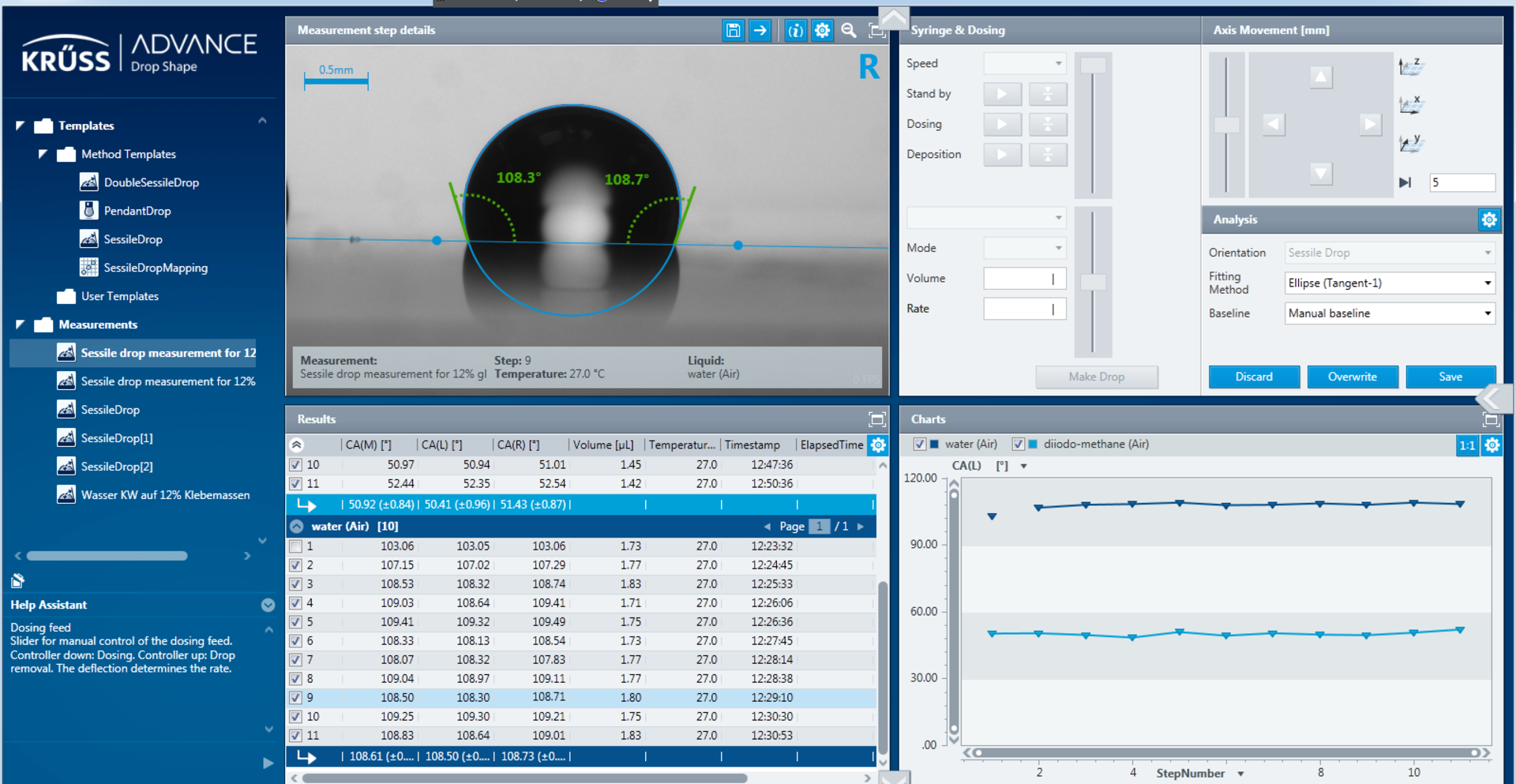
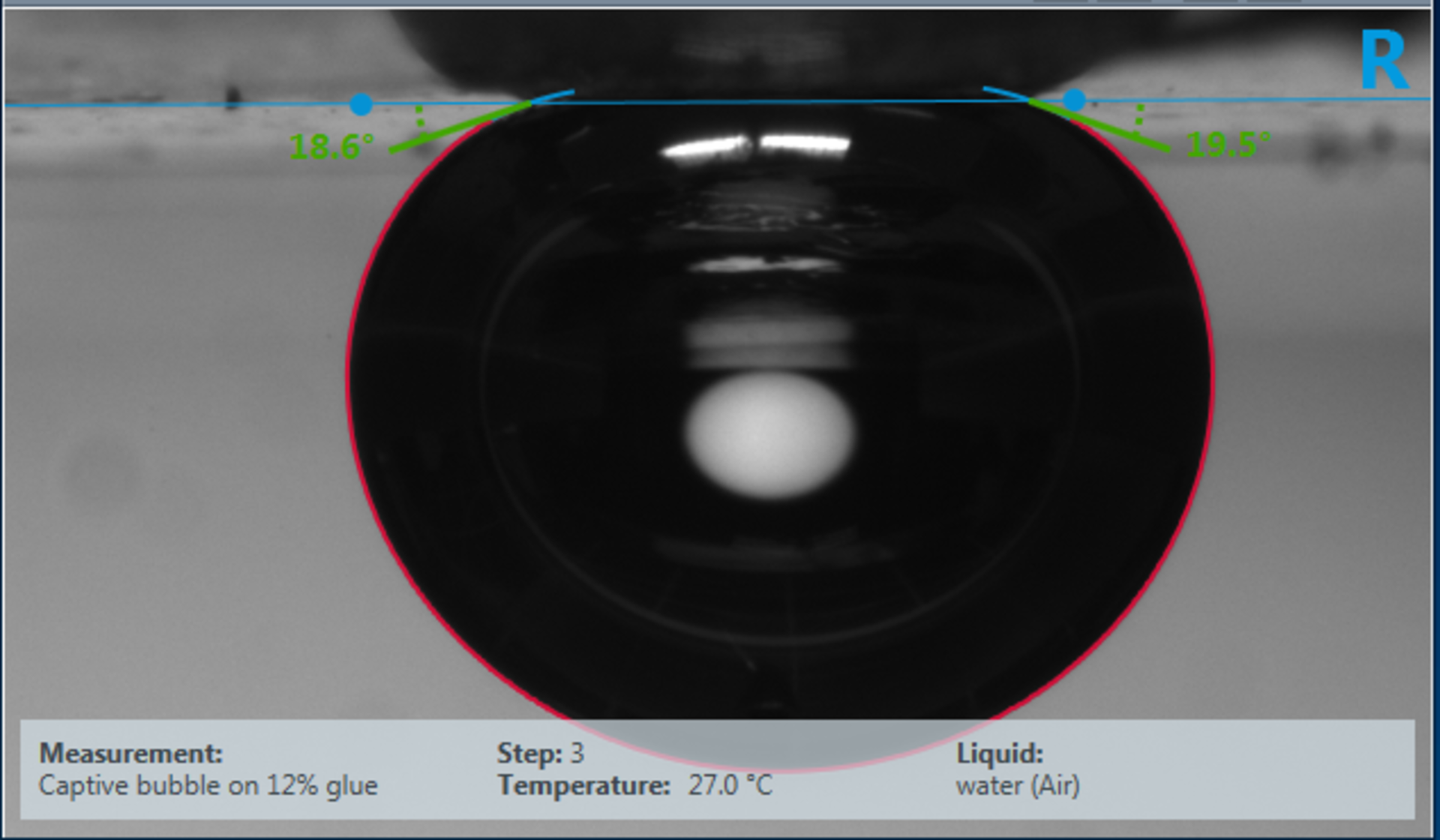
The static CA is 108° with a sessile drop, and 19° with a captive bubble. The static CA with a sessile drop describes the result of a wetting process, i.e. a previously non-wetted sample is wetted. As is to be expected, the measured sessile drop CA is comparable with the advancing CA according to Wilhelmy, which likewise depicts the wetting process. Conversely, the static CA with the captive bubble describes the result of a de-wetting process. Analogously, the CA is comparable with the receding CA according to Wilhelmy. Sessile drop and captive bubble produce a value for a kind of “static contact angle hysteresis” which, at 89°, is of a similar magnitude to the tensiometric Wilhelmy CA measurements. However, this method was not pursued further as captive bubble measurements are more elaborate experimentally, particularly when an averaging over larger sample areas comparable to the Wilhelmy method is required.
Relationship between water CA and acrylic acid content
The receding angles immediately after conditioning were the same for all samples within the limits of error scatter, and no clear trend for the receding angle as a function of the acrylic acid concentration was observed. The receding angles showed a greater scatter than the advancing angles, which would be explained by source effects of the PSA. Conversely however, the advancing angles were extremely reproducible. Figure 7 shows the water advancing angle as a function of the acrylic acid content samples straight after conditioning. Here, the measurement scatter is less than the diameter of the data points.
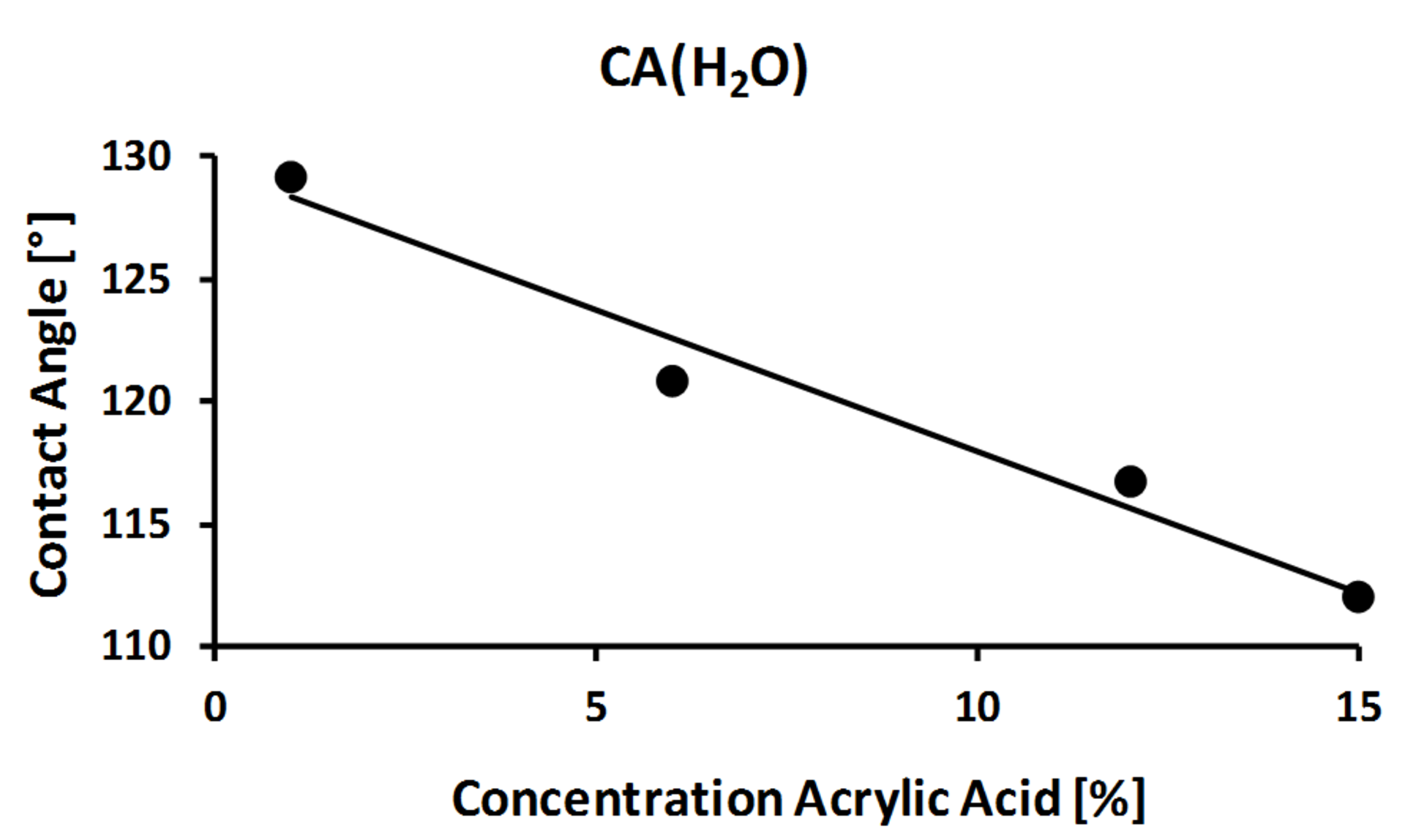
According to this, there is a significant linear relationship between acrylic acid content and the water advancing CA. In spite of the Hydrophobic Recovery effect, the characteristic therefore follows the tendency, which is to be expected due to increasing polarity, that the water advancing CA decreases with increasing acrylic acid content. Even when a large part of the polar side chains have presumably turned into the PSA and therefore do not fully affect the wetting process (very large CA >90°), the water CA is still sufficiently sensitive to quantitatively detect the acrylic acid content.
Conditioning in water appears to reduce the scatter of the CA within the samples. It would therefore be an interesting aspect for further studies to investigate whether the water CA in a second Wilhelmy measuring cycle on non-conditioned samples gives data comparable to Figure 7. It is also an interesting question for future investigations whether the captive bubble method, which unlike the Wilhelmy receding angle measurement is unaffected by possible swelling effects, can demonstrate a significant correlation between CA and acrylic acid content.
Summary
PSA with acrylic acid contents of 1 to 15% were conditioned in water for 72 h. Measurements of the water and diiodomethane contact angles (CA) on the samples showed no clear time-dependency within 170 hours of conditioning. This and the observance of highly evident hysteresis of the water CA measured using the Wilhelmy method and comparably large differences between optical sessile drop and captive bubble CA measurements indicate that a possible Hydrophobic Recovery of the samples takes place in a timescale of less than one minute.
Advancing angle measurements according to Wilhelmy with water show a significant and approximately linear relationship to the acrylic acid content of the PSA. The water CA reduces from 128° (1%) to 112° (15%) with increasing acrylic acid content. Compared with the formerly used optical CA measurement on a sessile drop, tensiometric CA measurement was judged to be a particularly advantageous measurement method for these samples.
It is distinguished in particular by better statistics (averaging over larger surfaces) and reproducibility, and is also often less dependent on the user than sessile drop measurements carried out manually.
Bibliography
- [1] C. Duc, A. Vlandas, G.G. Malliaras, and V. Senez, Soft Matter, 2016, 12, 5146-5153.
- [2] H. Yasuda, A. K. Sharma and T. Yasuda, J Polym. Sci Polym. Phys. Ed., 1981, 19, 1285.
- [3] Y. L. Chen, C. A. Helm and J. N. Israelachvili, J. Physcial Chem., 1991, 95, 10736–10747.
- [4] Rosa Di Mundo, Fabio Palumbo, Plasma Process. Polym. 2011, 8, 14–18, DOI: 10.1002/ppap.201000090.
This work has been done in close cooperation with tesa SE headquarters in Germany and all samples were kindly provided by tesa SE.
As mentioned in this application report, a K100 Force Tensiometer had been used for carrying out some of the measurements. The same measuring methods, only with improved precision and ease of use, can now be performed with the up-to-date Tensíío instrument.