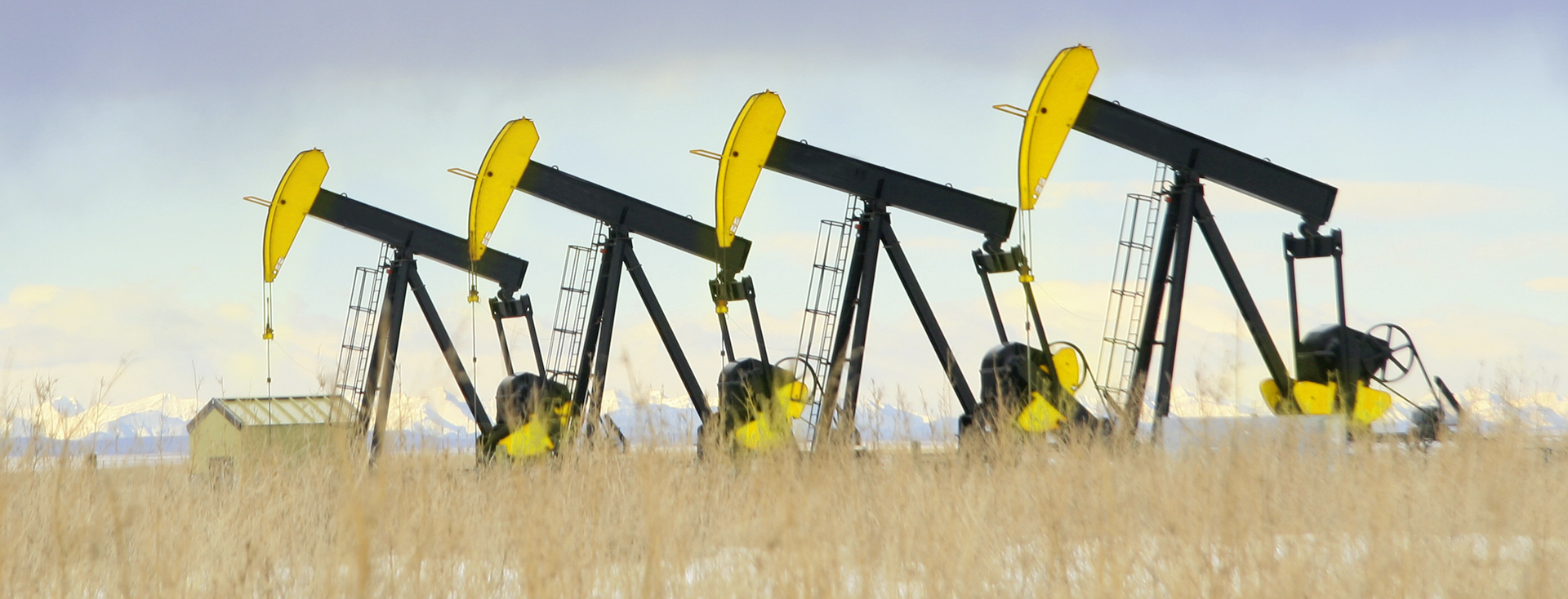
Foam stability and foam structure under high pressure for tertiary oil production
Foam analysis under pressure up to 35 MPa with a new measuring instrument
Foam properties such as stability, structure, foamability and bubble size distribution depend, among other things, on the type and phase behavior and concentration of the stabilizing surfactant, on the salt content as well as on additives such as polymers and finally on the temperature. When foams are used in tertiary oil production, a further factor occurs that hardly plays a role in conventional foam analyses: the pressure prevailing in a reservoir, which leads to a completely different foamability and above all stability behavior compared to standard conditions. The influence of this particularly important parameter has so far only been investigated to a limited extent due to a lack of available measurement technology.
With the High Pressure Foam Analyzer – HPFA, analyses of the formation, stability, and structure of liquid foams at up to 35 MPa and 120 °C have recently become possible in a single measurement. In this study, we present the first investigations on the pressure-dependent foaming behavior carried out with this instrument. The focus is on measurements of foam height and structure as well as time-dependent analyses of the relative foam height and mean bubble size of aqueous nitrogen foams. Reproducibility measurements provide well consistent results.
Furthermore, the influence of the gas type for the most important gases nitrogen (N2) and carbon dioxide (CO2) on the foam behavior was investigated.
Background
In order to use foams in tertiary oil production, the dispersion of gas bubbles in aqueous surfactant solution must be stable over a longer period of time and against the extreme conditions present in the deposit, such as high salinity, temperatures, and pressures. Before the multiphase flow in the pore space including all interactions with the rock matrix can be investigated, the behavior of the fluid phase (bulk foam) should be known. Depending on the surfactant-gas system used, pressure, temperature, various additives and interactions with salts and crude oils play a role.
Experimental part
Measuring instrument used
Since previous research work on foam structure and foam stability could only be carried out at atmospheric pressure and therefore the influence of the decisive parameter pressure could not be determined, the High Pressure Foam Analyzer – HPFA was developed as an apparatus for the scientific investigation of liquid foams under elevated pressures (pmax = 35 MPa) and temperatures (Tmax = 120 °C).
The core of the instrument is a high-pressure long-hole view cell in which incoming gas is led through a glass filter plate (frit) or a drill core into the aqueous surfactant solution above. Additives and/or crude oil can be injected into the aqueous surfactant solution or onto the foam with the aid of a connected mini-dosing unit before or during a measurement (see Fig. 1).
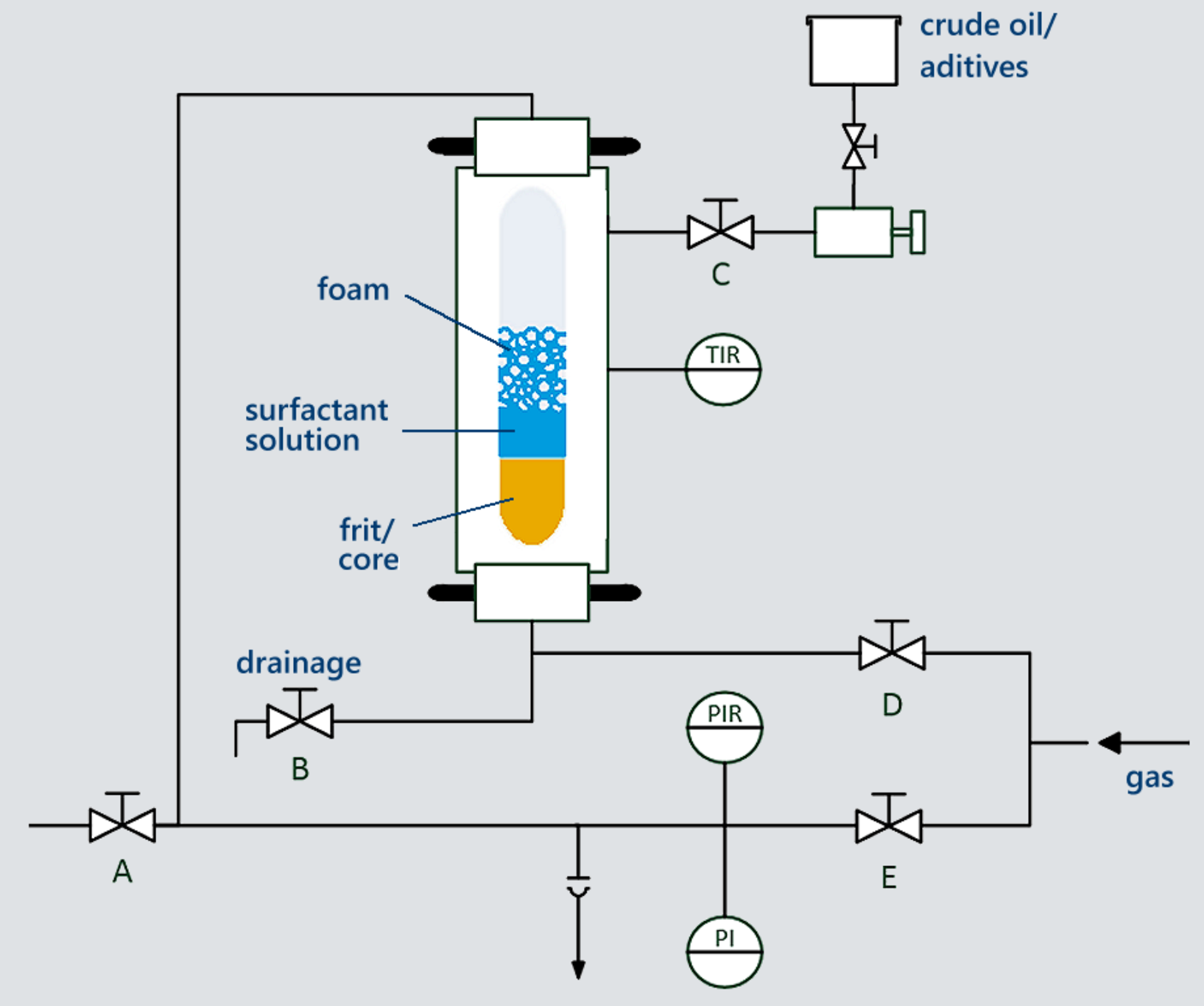
The gas entry as well as the pressure generation in the high pressure chamber is carried out by an additional pump (HP4790).
A continuous detection of the foam height is carried out optically with a camera using the transmitted light method. For the detection of the bubble size distribution, a special arrangement of two prisms is applied directly to the sight glass so that the foam structure can be recorded and evaluated (see Figs. 2 and 3).
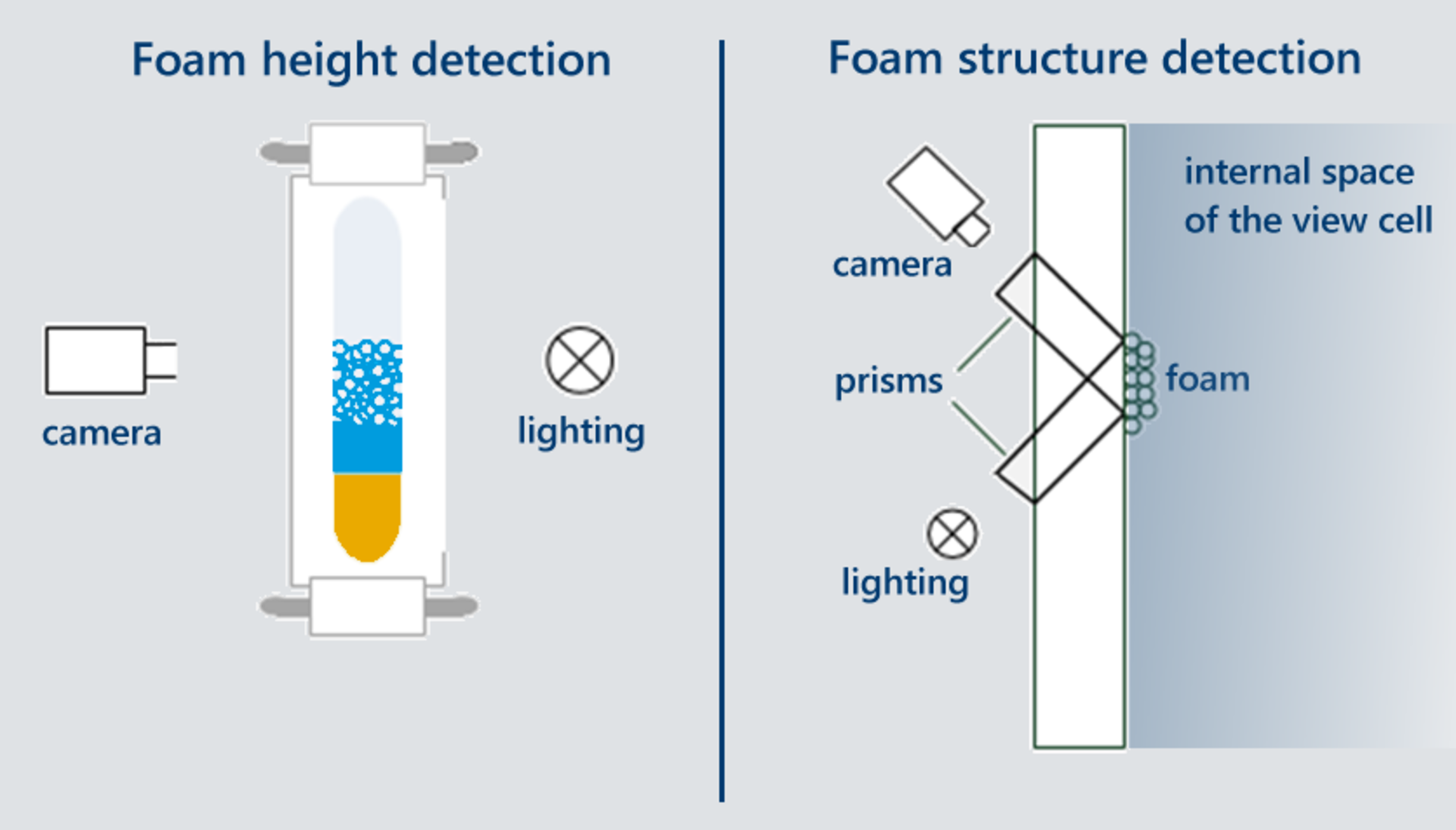
Using the ADVANCE software, the individual images of the foam height and foam structure are continuously recorded and evaluated. The actual bubble sizes are determined in real time and visualized in a histogram for each recorded point in time.
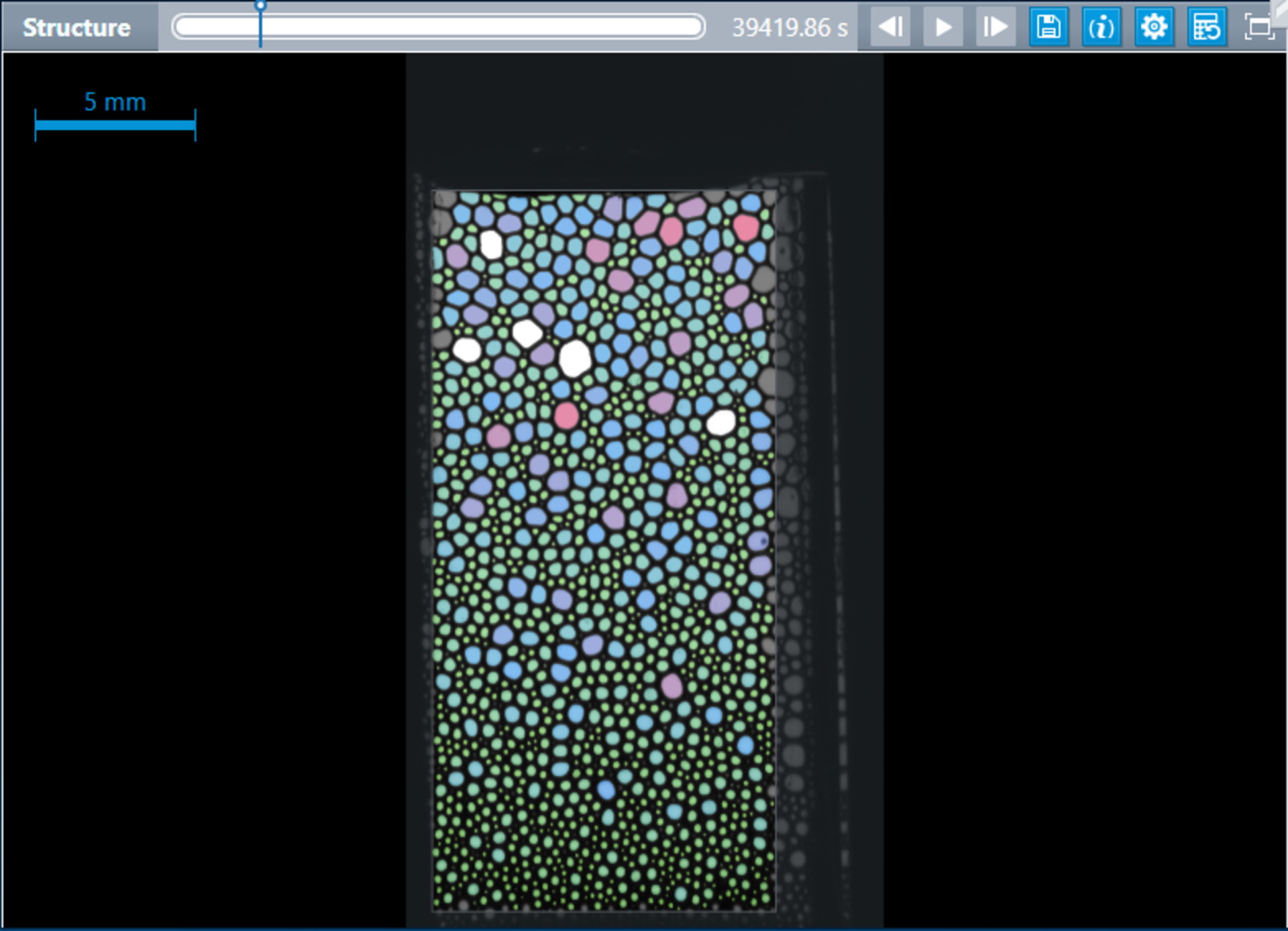
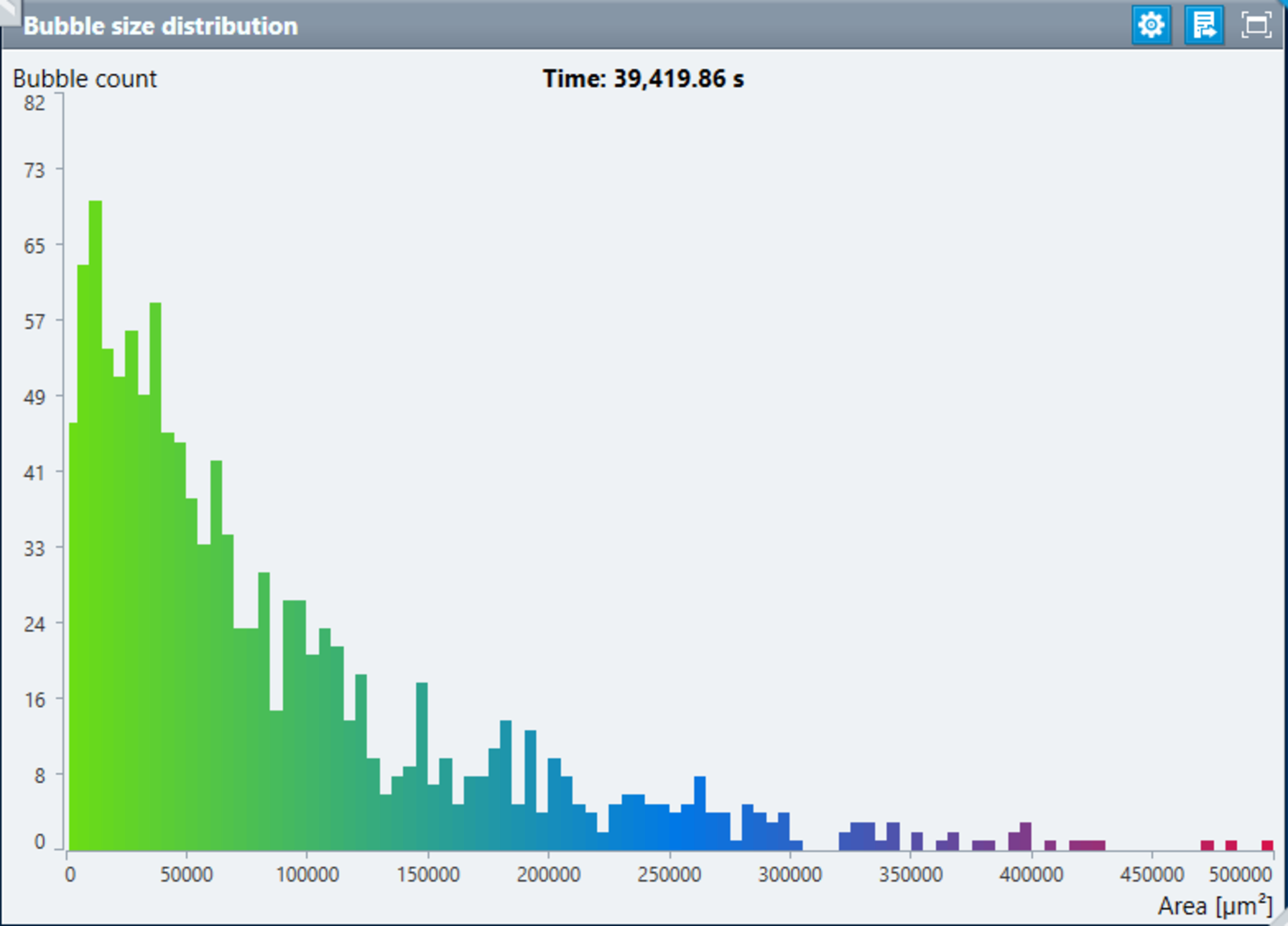
Data such as mean bubble size and statistical bubble size distribution are also available for each measurement time.
Examined samples and measurement conditions
The stability and structure of foams from an aqueous solution of an anionic surfactant were investigated. The surfactant samples were foamed with a gas volume flow of 50 mL/min and a gas volume of 100 mL through a frit with porosity G2 (pore size of 16 to 40 µm). As gases nitrogen (N2) and carbon dioxide (CO2) were used at a temperature of 45 °C and different pressures between atmospheric pressure and 15 MPa.
The change in foam height and foam structure, especially the mean bubble size, was analyzed after foaming over a period of up to 18 hours. The experimental conditions are summarized again in Table 1:
Temperature | 45 °C |
Surfactant | sodium-C14-16-Olefinsulfonat |
Surfactant concentration | 2 g/L in demin. H2O |
Introduced gas volume | 100 mL* |
Gas volume flow during foam generation | 50 mL/min |
Porosity of filter plate | G2 (16 to 40 µm) |
Gases used | N2, CO2 |
Table 1: Experimental conditions
Results
Pressure-dependent foam stability
When considering the foam stability of an aqueous N2 foam stabilized with an anionic surfactant, it can be seen that the stability increases with increasing pressure, with the most significant increase in stability being in the low pressure range (see Fig. 4). In the observation period of 18 hours no complete decomposition has taken place under increased pressures, so that one can speak of extremely stable foams.
With regard to the mean bubble size, the largest differences can also be found at low pressures. For example, the initial mean bubble size of a foam produced at ambient pressure differs significantly from the initial mean bubble sizes at higher pressures of 5 to 15 MPa. The mean bubble size increases with time at all pressures; this effect decreases with increasing pressures and is hardly noticeable at 15 MPa. Here the bubble size is very constant, which also indicates a high stability of the foam.
The density and viscosity of the disperse (gas) phase and the surrounding (liquid) phase play a role in foam stability. With increasing pressure both the density and the viscosity of the nitrogen and the aqueous phase increase, whereby foams are stabilized with increasing pressure. The mobility of the surfactant molecules increases with increasing pressure so that Marangoni convection, which causes the liquid to flow from areas with lower surface tension to areas with higher surface tension, is accelerated under increased pressures. This stabilizes the foam lamellas. A possible destabilizing effect, namely the increasing gas solubility with the pressure, obviously plays a subordinate role in the foams formed with nitrogen.
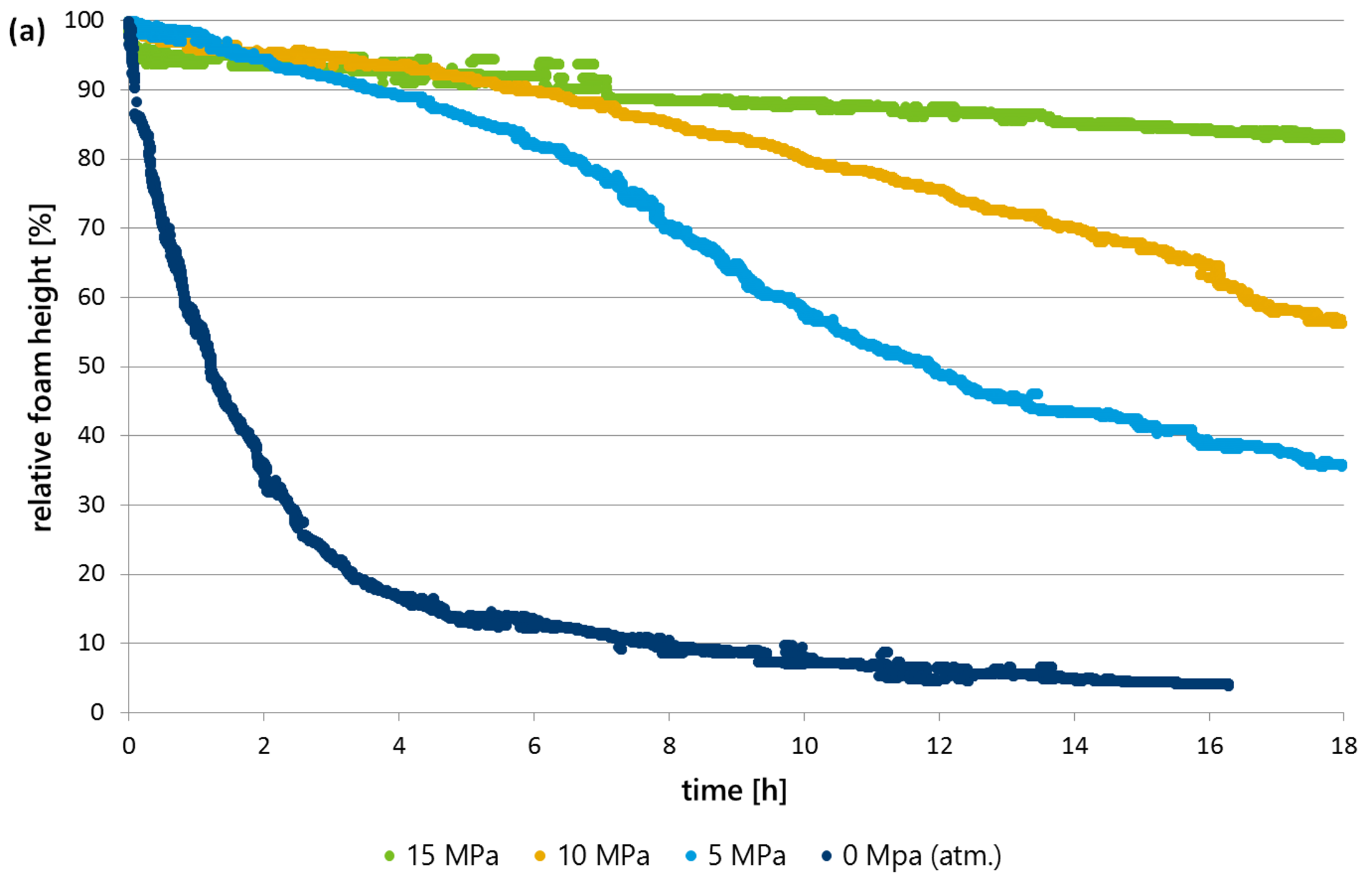
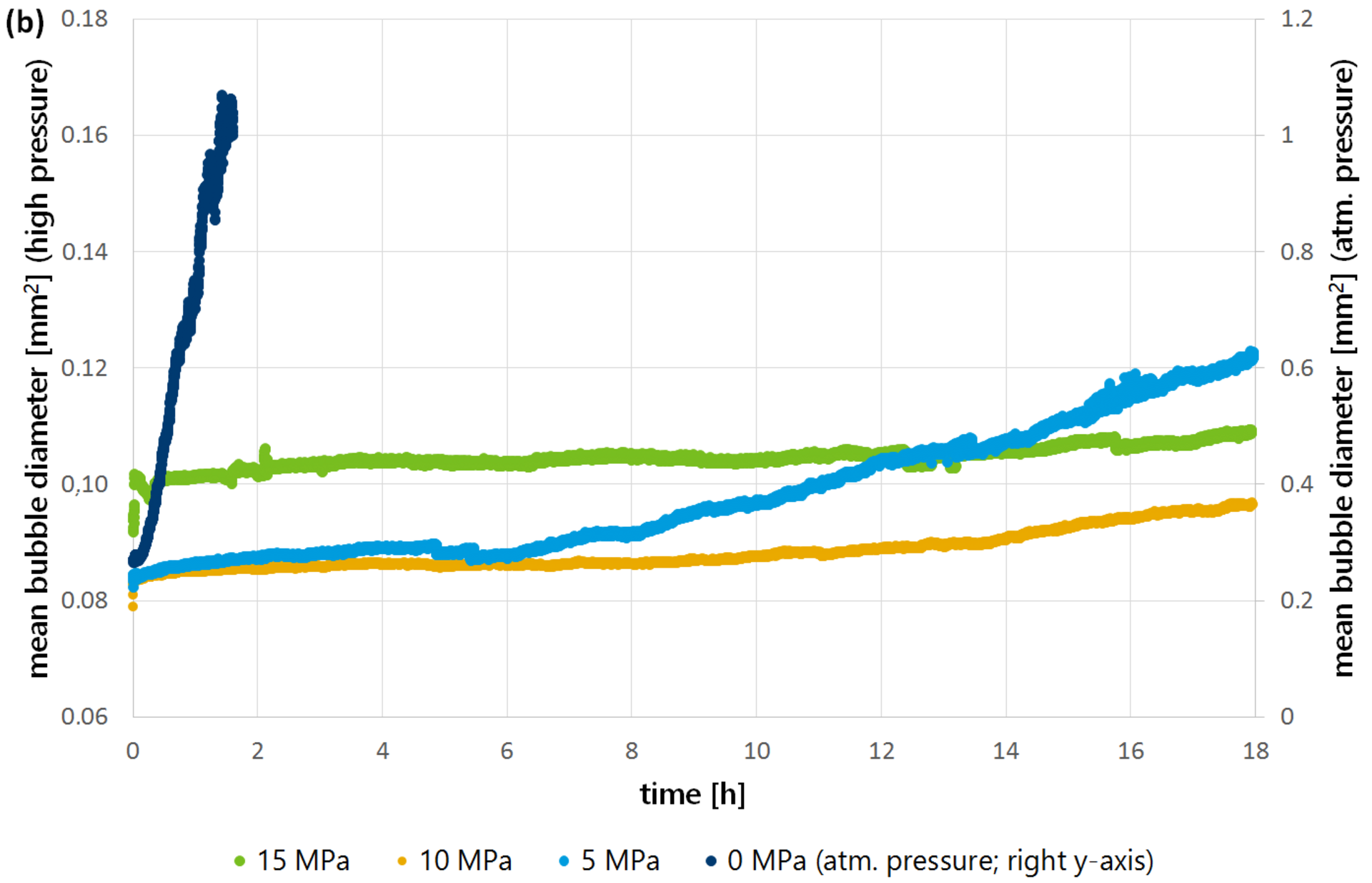
Gas-dependent foam stability
The foam stability and the development of the mean bubble size over the lifetime of a foam depend on various physical parameters, some of which are highly pressure-dependent. These include, in particular, the interfacial tension between the two phases present in the foam, the density and viscosity, which vary over time due to material transfer, and the related mutual solubility.
Due to the fundamentally different substance properties of CO2 compared to N2 in the entire pressure range up to 35 MPa, the picture is completely different for foams formed with CO2 than for N2 foams. Fig. 5 shows the comparison between a foam formed with N2 and a foam formed with CO2 at a pressure of 5 MPa each. The other test conditions such as gas volume, surfactant type and concentration are identical (see Table 1).
The relative foam height of the CO2 foam decreases significantly faster than that of the N2 foam and is already below 10% after 2 hours (see Fig. 5a). CO2 foams disintegrate faster and are therefore more unstable than N2 foams.
The initial mean bubble size is comparable and therefore only pressure-specific and not gas-specific. The mean bubble size of CO2 foam increases significantly faster over time than that of N2 foamed samples (see Fig. 5 b).
The half-life is approximately 12 hours when using N2 and only about 50 minutes when using CO2.
The decisive factor for this could be the increasing mutual solubility of CO2 and aqueous surfactant solution, especially with increasing pressure. In particular, the increased material transfer of CO2 in and through the foam lamellae leads to a faster increase in the average bubble size (Ostwald ripening) and thus to reduced foam stability.
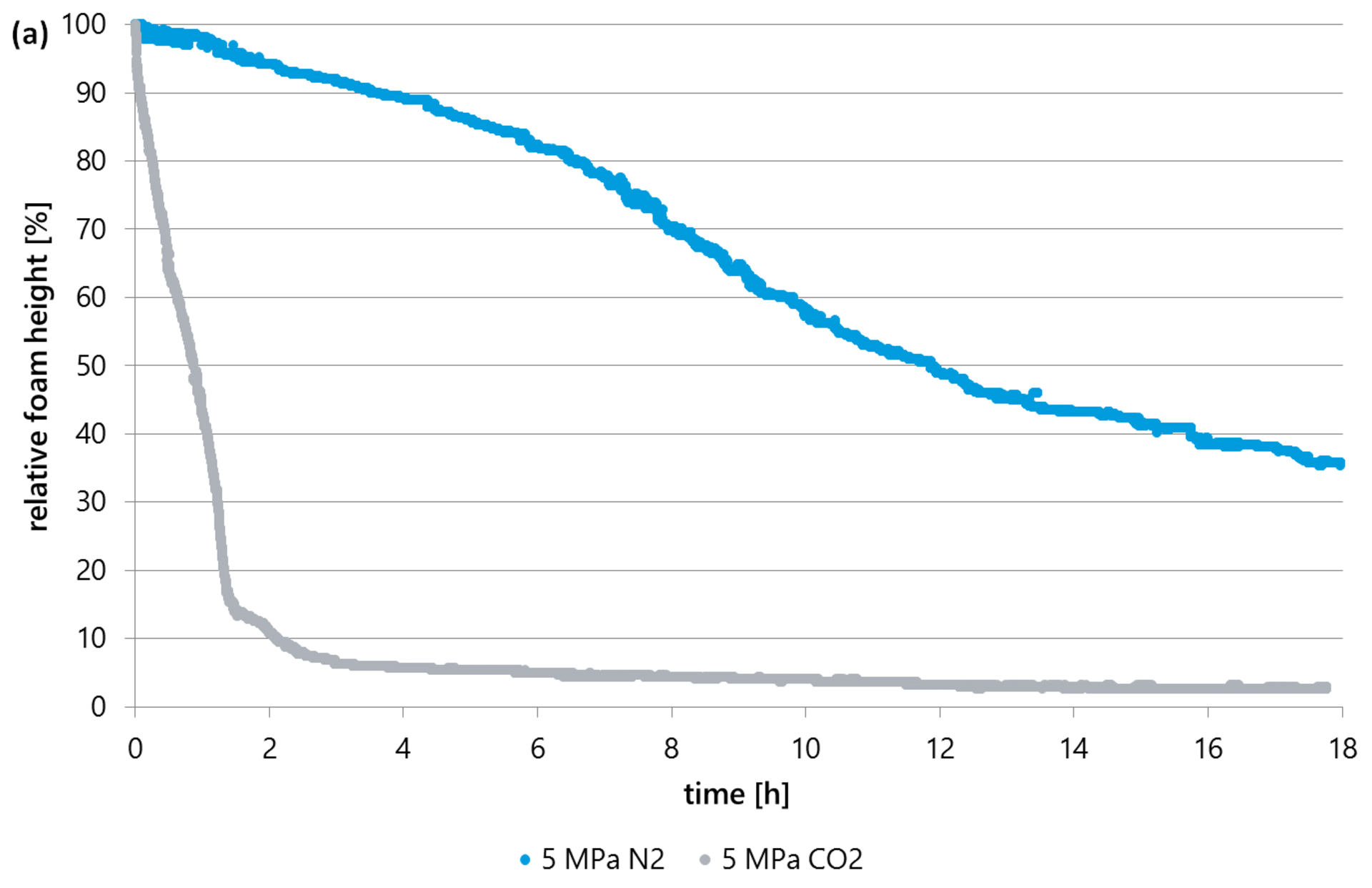
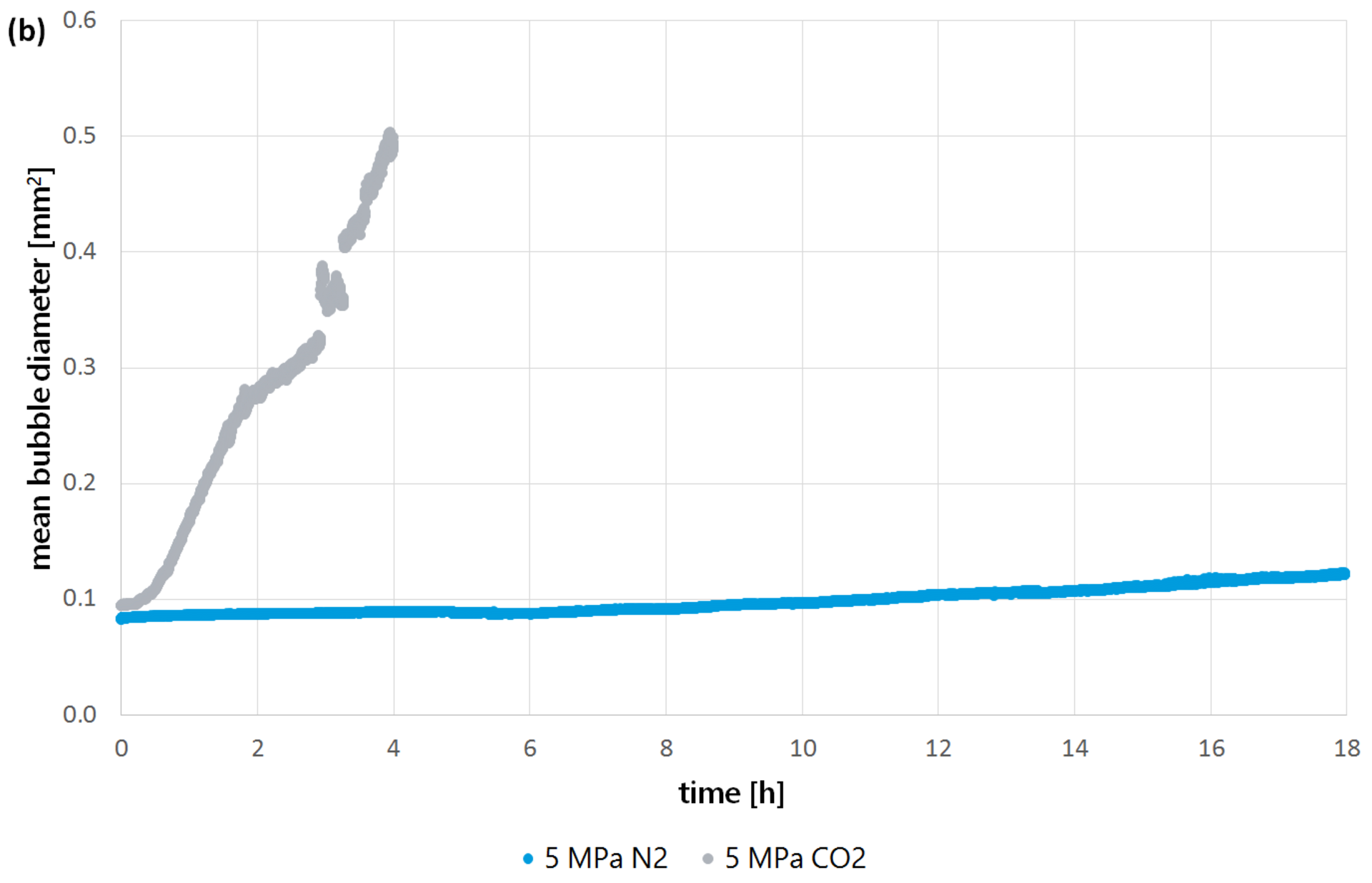
Reproducibility
Since foams are very complex systems, reproducibility is a major challenge. The deviation between measurements under the same test conditions increases with increasing measurement duration. Fig. 6 shows two measurements under identical conditions at a pressure of 15 MPa. The results are easily reproducible. The deviation of the two measurements after 18 hours is 9% for the relative foam height and 1% for the medium bubble size.
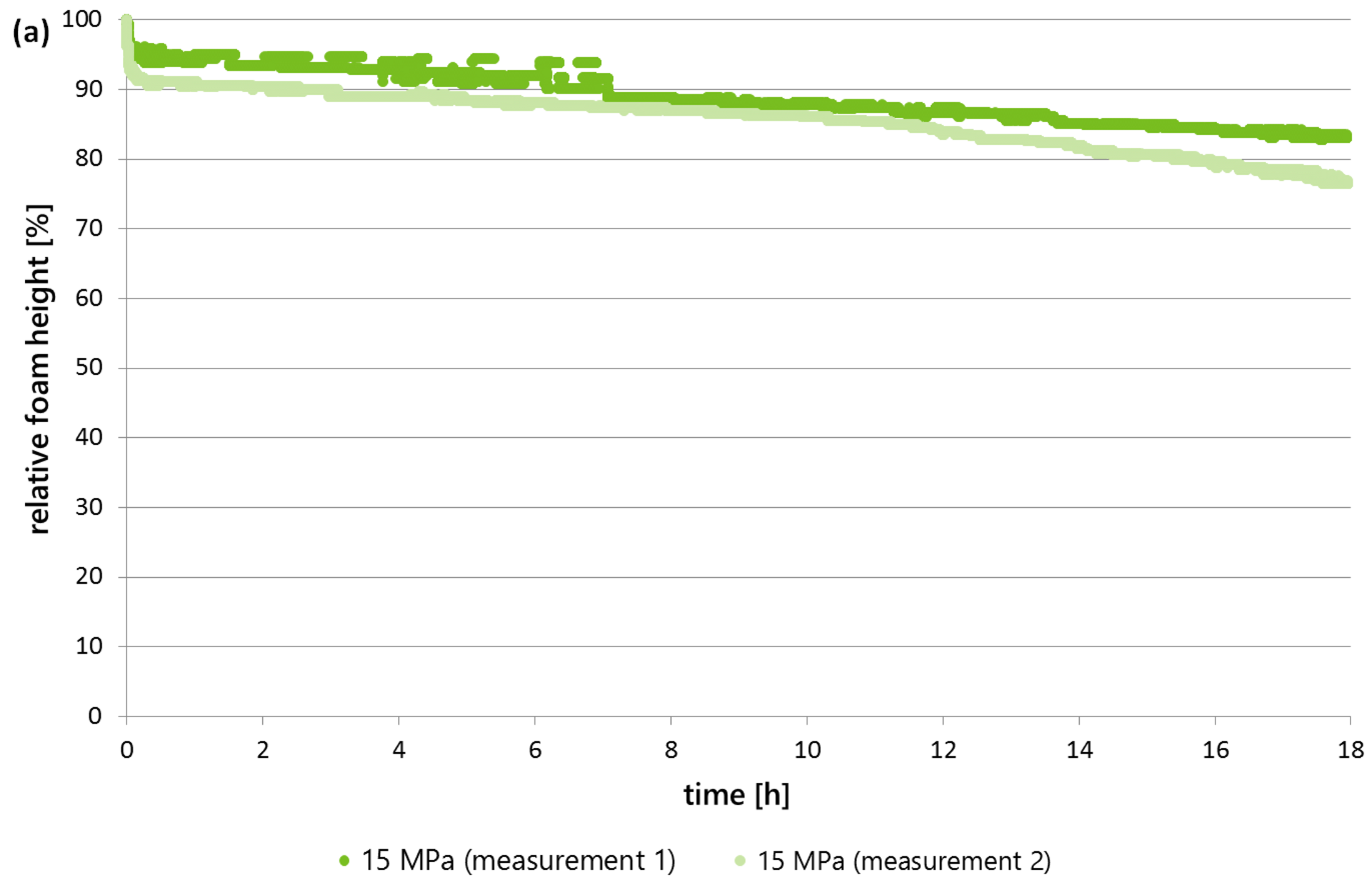
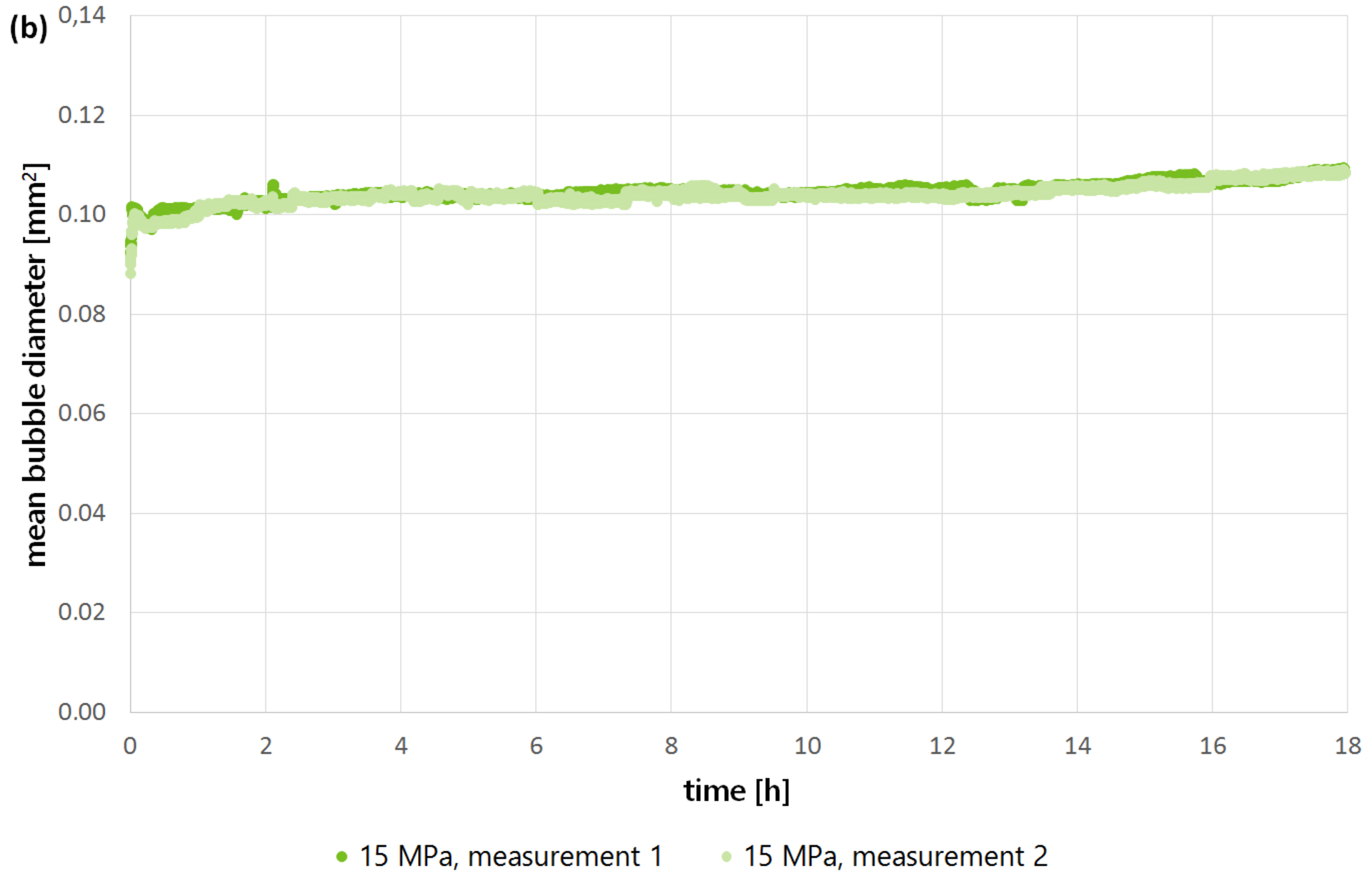
Summary
First investigations of aqueous foams under high pressures with the High Pressure Foam Analyzer – HPFA show the considerable influence of an increase in pressure on the foam properties. The relative foam height and the mean bubble size of aqueous surfactant-stabilized N2 foams are significantly more constant at high pressures than at lower pressures. The foam stability increases with increasing pressure, which can be explained by increased Marangoni convection at high pressures.
A comparison of foams formed with N2 and CO2 shows the influence of the gas type and the associated pressure-dependent material properties on the foam parameters. CO2 foams are significantly more unstable than N2 foams, which can be explained by the mutual solubility of CO2 and sample.
By means of exemplary repeat measurements a good reproducibility could be shown in the foam height analysis and an excellent repeat accuracy in the mean bubble diameter.
The new measuring technique and the strong influence of the pressure on the foam behaviour shown with its help opens up a whole field of promising research work with the help of which the use of foam in tertiary oil production can be optimized.
Bibliography
- M. Szabries, P Jaeger, Moh’d M. Amro: Foam Analysis at Elevated Pressures for Enhanced Oil Recovery Applications. Energy Fuels 2019,33, 5, 3743-3752. https://doi.org/10.1021/acs.energyfuels.8b03088
- P. Jaeger, M. Szabries, S. Bäßler, M. Jönsson, T. Schörck , A. Kordts: Foams at Elevated Pressure in EOR. An Innovative Method to Analyze Foam Stability and Structure. IBP1026 18, Rio Oil & Gas Expo and Conference 2018.